|
Research Interests |
|
|
We are interested in membrane biophysics and focus on 3 projects:
|
|
Chiral Sum Frequency Generation Spectroscopy-Biomolecular Interactions at Interfaces |
|
|
Aggregation of hIAPP. (A) The time-dependent chiral SFG spectra in the vibrational regions of N-H stretch (left) and amide I (right) after addition of DPPG. (B) The intensity of the N-H stretch and amide I signals as a function of time. Results of triplicate experiments are shown. (C) The aggregation model of hIAPP on a membrane surface as observed in the SFG experiments: adsorption as a random coil leads to formation of α-helical intermediates, which are converted to beta-sheet aggregates. (Adapted from Li et al. JACS 2011) |
Characterization of protein secondary structures using vibrational spectroscopy is challenging because of strong vibrational backgrounds from water and spectral overlapping of vibrational bands of various protein secondary structures. Our recent studies have shown that chiral sum vibrational frequency generation (SFG) spectroscopy can be used to address the challenge. We found that chiral SFG spectra of the amide I and N-H stretch of protein backbones provide highly characteristic vibrational signatures to distinguish parallel beta-sheets, anti-parallel beta-sheets, alpha-helices, 3-10 helices, and random-coils at interfaces. Because chiral SFG spectra are muted to achiral solvent, chiral SFG can be used to characterize chiral secondary structures at interfaces with zero water background. Using chiral SFG, we studied the aggregation of human islet amyloid polypeptide (hIAPP), which is associated with type II diabetes. We observed in situ and in real time the misfolding of hIAPP from disordered structures to alpha-helices and then beta-sheets on membrane surfaces. The results provide insight into the pathogenic mechanism for type II diabetes and guide rational design of drugs targeting the intermediates at the early stages of amyloid aggregation on membrane surfaces. The studies also demonstrate the general capacity of chiral SFG in probing self-assembling process of chiral structures at interfaces in situ and in real time. This development is useful in solving fundamental and engineering problems in biological and biomedical sciences.
References:
- Yan, E. C. Y.* Fu, L.; Wang, Z.; Liu, W. "Biological Macromolecules at Interfaces Probed by Chiral Sum Frequency Generation Spectroscopy" Special Thematic Issue: Aqueous Interfaces, Chem. Rev., 2014.
- Fu, L.; Wang, Z.; Yan, E.C.Y.* "N-H Stretching Modes Around 3300 Wavenumber From Peptide Backbones Observed by Chiral Sum Frequency Generation Vibrational Spectroscopy" Chirality, 2014.
- Liu, W.; Wang, Z.; Fu, L.; Leblanc, R. M.; Yan, E. C. Y. "Lipid Compositions Modulate Fluidity and Stability of Bilayers: Characterization by Surface Pressure and Sum Frequency Generation Spectroscopy", Langmuir, 29, 15022, 2013.
- Wang, Z.; Fu, L.; Yan, E.C.Y.* "C-H Stretch for Probing Self-Assembly of LK7beta into Chiral Macromolecular Structures at the Air-Water Interface by Chiral Sum Frequency Generation Spectroscopy"Langmuir 29, 4077, 2013
- Fu, L.; Xiao, D.Q.; Wang, Z.; Batista, V.S.*; Yan, E.C.Y.* “Chiral Sum Frequency Generation for Probing the Hydrogen/Deuterium Exchange in Antiparallel beta-Sheet Peptides at Interfaces” J. Am. Chem. Soc. 135, 3592,2013
- Xiao, D.; Fu, L.; Liu, J.; Batista, V.S.*; Yan, E.C.Y.* “Amphiphilic Adsorption of Human Islet Amyloid Polypeptide Aggregates to Lipid/Aqueous Interfaces” J. Mol. Biol., 421, 537, 2012
- Fu, L.; Wang, Z.; Yan, E.C.Y.* “Chiral Vibrational Structures of Proteins at Interfaces Probe by Sum Frequency Generation Spectroscopy” Invited Submission, Special Issue: Applications of Circular Dichroism, Int. J. Mol. Sci., 12, 9404,2011
- Fu, L.; Liu, J.; Yan, E.C.Y.* “Chiral Sum Frequency Generation for Identification of Protein Secondary Structures at Interfaces” J. Am. Chem. Soc. 133, 8094, 2011
- Fu, L.; Ma, G.; Yan, E.C.Y.* “In Situ Misfolding of Human Islet Amyloid Polypeptide at Interfaces Probed by Sum Frequency Generation Spectroscopy” J. Am. Chem. Soc. 132, 5405, 2010
- Ma, G.; Liu, J.; Fu, L.; Yan, E.C.Y.* “A Vibrational Sum Frequency Generation Spectrometer with Broad Bandwidth Technology” Appl. Spectro., 63, 528,2009
Back to the top
|
|
Signal Transduction across Biomembranes-G Protein-Coupled Receptors |
|
|
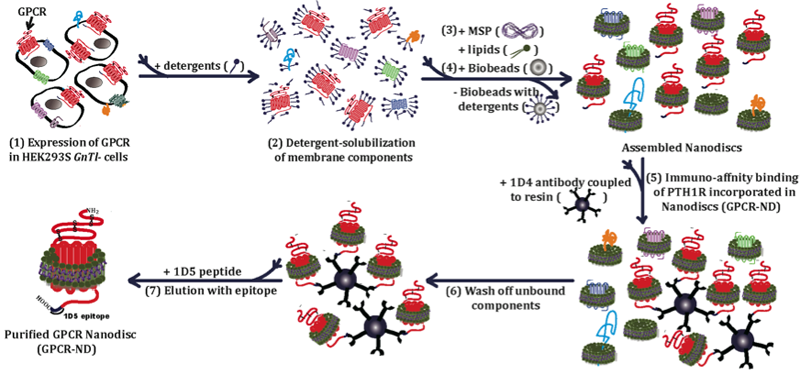
Schematic of purification of a family B GPCR in nanodiscs. The purification procedure includes (1) expression of a family B GPCR in a mammalian expression system, (2) solubilization of membrane components of the expression system using detergent, (3) incubation of solubilized membrane components with phospholipid and the membrane scaffold protein, MSP1E3D1 (4) addition of Bio-Beads to remove detergent by physical adsorption to induce formation of nanodiscs containing membrane proteins, (5) immuno-affinity binding of the GPCR incorporated in nanodiscs (GPCR-ND) to antibody-conjugated resin, (6) washing resins bound with GPCR-ND, and (7) elution with epitope to yield purified GPCR-ND. (Adapted from Mitra et al. ACS Chem Bio 2013) |
We focus on 7-alpha-helical transmembrane G protein-coupled receptors (GPCRs) that belong to the largest gene family in the human genome and represent important drug targets. We develop method to purify GPCRs using nanoscale lipid bilayer disc particles (nanodiscs). We focus on family B GPCRs, which is an important family in endocrinology, in particular, parathyroid hormone 1 receptor and glucagon like peptide receptor, which are drug targets for osteoporosis and diabetes, respectively. In addition, we apply unnatural amino acid mutagenesis to site-specifically incorporate spectroscopic probes into GPCRs. Because purification of GPCRs in the quality and quantity that allows quantitative biophysical studies is one of the biggest challenges, this approach could provide a breakthrough to apply biophysical spectroscopy to obtain highly selective spectroscopic readout to report conformational changes of GPCRs during activation. The results will reveal the activation mechanism of GPCRs at the molecular level, guiding rational drug design targeting GPCRs for developing new therapeutics.
References:
- Mitra, N.; Liu, Y.; Liu, J.; Serebryany, E.; Mooney, V.; DeVree, B.T.; Sunahara, R.; Yan, E.C.Y.* “Calcium Dependent Ligand Binding and G-protein Signaling of Family B GPCR Parathyroid Hormone 1 Receptor Purified in Nanodiscs” ACS Chem. Biol. 8, 617, 2013
- Zhu, G.A.; Serebryany, E.; Yan, E.C.Y.* “Rational Design of Supramolecular Lipid/Detergent Assemblies for Purification of G Protein-Coupled Receptors” Encyclopedia of Supramolecular Chemistry,2012
- Serebryany, E.; Zhu, G.F.; Fu, L.; Liu, J.; Yan, E.C.Y.* “Artificial Membrane-like Environments for In Vitro Studies of Purified G-protein Coupled Receptors” Invited Review, BBA-Biomembrane, 1818, 225, 2011
- Ye, S.; Köhrer, C.; Huber, T.; Kazmi, M.; Yan, E.C.Y.; Sachdev, P.; Bhagat, A.; RajBhandary, U.L.; Sakmar, T.P. “Site-specific Incorporation of Keto Amino Acids Into Functional G Protein-Coupled Receptors Using Unnatural Amino Acid Mutagenesis” J. Biol. Chem. 283, 1525, 2008
Back to the top |
|
|
Molecular Mechanism of Vision-Biophysical Studies of Visual Pigments |
|
|
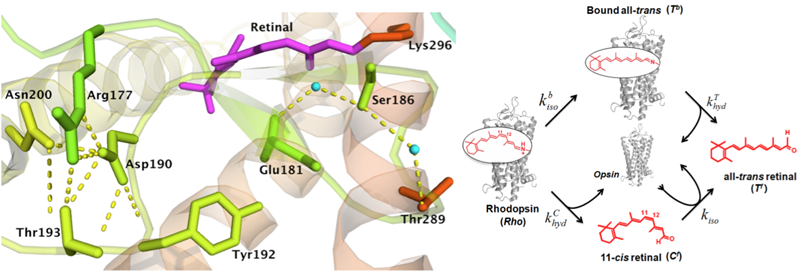
Crystal structure of rhodopsin and kinetic model of the thermal processes. The upper figure shows a hydrogen bonding network at the retinyl binding site. The lower figure show the two pathways of thermal reactions of rhodospin. First, dark-state rhodopsin undergoes thermal isomerization to yield all-trans retinal bound to opsin via an unprotonated Schiff base (Tb) – a Meta II-like state. The Schiff base in Tb breaks to yield all-trans retinal free in solution (Tf). Second, rhodopsin undergoes hydrolysis of the protonated Schiff base to form 11-cis retinal free in solution (Cf), along with opsin protein. Then Cf isomerizes in the presence of opsin protein to yield Tf. (Adapted from Liu et al 2013) |
GPCR rhodopsin is an extremely sensitive biological light detector. It contains the 11-cis retinyl chromophore. Light triggers photoisomerization in the chromophore to generate visual signal. In the dark, the chromophore can very occasionally overcome the energy barrier to undergo thermal isomerization, producing the same physiological response to generates false signal that is dark noise. The rate of thermal isomerization in rhodopsin is extremely low with a half-life of 420 years! This keeps the dark extremely noise low for reliable dim-light detection. Thus, the molecular origin of low dark noise is the key to understanding the mechanism of dim-light vision. However, it remains elusive. Using site-directed mutagenesis and studying reactions kinetics, we investigate the role of hydrogen bonds in suppressing dark noise in rhodopsin. The studies can provide a strong fundamental basis for understanding inherited eye diseases, such as retinitis pigmentosa, caused by mutations in the rhodopsin gene, and molecular evolution of vision in vertebrates.
References:
- Guo, Y.; Skharan, S.; Liu, J.; Liu, M. Y.; Batista, V. S.; Tully, J. C.; Yan, E. C. Y.* "Role of Unusual Temperature-Dependent Kinetics of Thermal Reactions of Rhodopsin in Vertebrate Dim-Light Vision" Proc. Natl. Acad. Sci. U.S.A. 108, 26111, 2014.
- Sekharan, S.*; Mooney, M.L.; Rivalta, I.; Kazmi, M.A.; Neitz, M.; Neitz, J.; Sakmar, T.P.; Yan, E.C.Y.*, Batista, V.B.* "Spectral Tuning of Ultraviolet Cone Pigments: An Interhelical Lock Mechanism" J. Am. Chem. Soc. 135 (51), 19064, 2013.
- Liu, M.Y.; Liu, J.; Mehrotra, D.; Liu, Y.; Guo, Y.; Baldera-Aguayo, P.A.; Mooney, V.; Nour, A.M.; Yan, E.C.Y.* “Thermal Stability of Rhodopsin and Progression of Retinitis Pigmentosa: A Comparison of S186W and D190N Rhodopsin Mutants” J. Biol. Chem., 8, 671, 2013
- Mooney, V.L.; Szundi, I.; Lewis, J.W.; Yan, E.C.Y.*; Kliger, D.S.* “Schiff base protonation changes in siberian hamster ultraviolet cone pigment photointermediates” Biochemistry, 51, 2603, 2012
- Liu, J.; Liu, M.; Fu, L.; Yan, E.C.Y.* “Chemical Kinetic Analysis of Thermal Decay of Rhodopsin Reveals Unusual Energetics of Thermal Isomerization and Hydrolysis of Schiff Base" J. Biol. Chem. 286, 38408, 2011
- Liu, J.; Liu, M.Y.; Mooney, V.; Bhagat, A.; Nguyen, J; Yan, E.C.Y.* "Thermal Properties of Rhodopsin: Insight into Molecular Mechanism of Dim-Light Vision" J. Biol. Chem. 286, 27622,2011
- Liu, J; Liu, M.Y.; Nguyen, J.B.; Bhagat A.; Mooney, V.; Yan, E.C.Y.* “Thermal Decay of Rhodopsin: Role of Hydrogen Bonds in Thermal Isomerization of 11-cis Retinal in the Binding Site and Hydrolysis of Protonated Schiff Base” J. Am. Chem. Soc. 131, 8750, 2009
Back to the top
|
|
|
|
|
|
Updated Jun. 2014 |
|